The Contribution of Dispersion to the Intrinsic Energy Barriers of Neutral Model Diels-Alder Reactions
DOI:
https://doi.org/10.29356/jmcs.v68i1.1867Keywords:
Diels-Alder reaction, Activation barrier, Intrinsic reaction coordinate, Dispersion interactions, Distortion/interaction modelAbstract
The intrinsic reaction coordinates for the cycloadditions between ethene and 1,3-butadiene, and ethene and cyclopentadiene, were determined at the SCS-MP2/aug-cc-pVTZ level of theory. The energy contents of the points determined for both coordinates were decomposed into their deformation and interaction contributions. From this analysis it is concluded that the higher reaction barrier for the butadiene-ethene cycloaddition can be attributed primarily to the conformational change of butadiene required by the reaction (higher deformation energy). There is also a minor contribution of the interaction term, which is more stabilizing for the cyclopentadiene-ethene reaction. An additional decomposition of these terms into their Hartree-Fock and SCS-MP2 correlation components suggests that the higher stabilization of the transition state of the cyclopentadiene-ethene cycloaddition is mostly due to stronger dispersion interactions between reactants, resulting from the larger contact surface between them, and not to stabilizing electronic effects.
Resumen. Se determinaron las coordenadas intrínsecas de reacción para las cicloadiciones entre eteno y 1,3-butadieno, y eteno y ciclopentadieno al nivel de teoría SCS-MP2/aug-cc-pVTZ. La energía de los puntos obtenidos en ambas coordenadas se descompuso en sus contribuciones de deformación e interacción. A partir de este análisis se concluye que la mayor barrera energética para la cicloadición eteno-butadieno puede atribuirse, principalmente, al cambio conformacional del butadieno requerido por la reacción (mayor energía de deformación). También se encuentra que el término de interacción es más estabilizante para la reacción entre ciclopentadieno y eteno, aunque la contribución de este término es menor. La descomposición adicional de las energías de interacción de estas reacciones en sus componentes de Hartree-Fock y de correlación SCS-MP2, sugiere que la mayor estabilización del estado de transición en la reacción entre ciclopentadieno y eteno, se debe principalmente a la interacción de dispersión más fuertemente estabilizante entre estos reactantes, resultado de la mayor superficie de contacto entre ellos y no a efectos electrónicos estabilizantes.
Downloads
References
Hoffmann, R.; Woodward, R. B. J. Am. Chem. Soc. 1965, 87, 4388-4389. DOI: https://doi.org/10.1021/ja00947a033. DOI: https://doi.org/10.1021/ja00947a033
Hoffmann, R.; Woodward, R. B. Acc. Chem. Res. 1968, 1, 17-22. DOI: https://doi.org/10.1021/ar50001a003. DOI: https://doi.org/10.1021/ar50001a003
Woodward, R. B.; Hoffmann, R., The Conservation of Orbital Symmetry. Academic Press: New York, 1970. DOI: https://doi.org/10.1016/B978-1-4832-3290-4.50006-4
Fukui, K.; Yonezawa, T.; Shingu, H. J. Chem. Phys. 1952, 20, 722-725. DOI: https://doi.org/10.1063/1.1700523. DOI: https://doi.org/10.1063/1.1700523
Fukui, K. Acc. Chem. Res. 1971, 4, 57-64. DOI: https://doi.org/10.1021/ar50038a003. DOI: https://doi.org/10.1021/ar50038a003
Houk, K. N. Acc. Chem. Res. 1975, 8, 361-369. DOI: https://doi.org/10.1021/ar50095a001. DOI: https://doi.org/10.1021/ar50095a001
Fleming, I. Frontier Orbitals and Organic Chemical Reactions. John Wiley & Sons: Chichester, 1976.
Robiette, R.; Marchand-Brynaert, J.; Peeters, D. J. Org. Chem. 2002, 67, 6823-6826. DOI: https://doi.org/10.1021/jo025796u. DOI: https://doi.org/10.1021/jo025796u
Domingo, L. R.; Aurell, M. J.; Pérez, P.; Contreras, R. Tetrahedron. 2002, 58, 4417-4423. DOI: https://doi.org/10.1016/S0040-4020(02)00410-6. DOI: https://doi.org/10.1016/S0040-4020(02)00410-6
Domingo, L. R.; Saez, J. A. Org. Biomol. Chem. 2009, 7, 3576-3583. DOI: https://doi.org/10.1039/B909611F. DOI: https://doi.org/10.1039/b909611f
Domingo, L. R.; Chamorro, E.; Perez, P. Org. Biomol. Chem. 2010, 8, 5495-5504. DOI: https://doi.org/10.1039/C0OB00563K. DOI: https://doi.org/10.1039/c0ob00563k
Sauer, J.; Lang, D.; Mielert, A. Angew. Chem., Int. Ed. 1962, 1, 268-269. DOI: https://doi.org/10.1002/anie.196202683. DOI: https://doi.org/10.1002/anie.196202683
Carey, F. A.; Sundberg, R. J. Advanced Organic Chemistry Part A: Structure and Mechanism. Fifth ed.; Springer: New York, 2007.
Bartlett, P. D.; Schueller, K. E. J. Am. Chem. Soc. 1968, 90, 6071-6077. DOI: https://doi.org/10.1021/ja01024a024. DOI: https://doi.org/10.1021/ja01024a024
Joshel, L. M.; Butz, L. W. J. Am. Chem. Soc. 1941, 63, 3350-3351. DOI: https://doi.org/10.1021/ja01857a033. DOI: https://doi.org/10.1021/ja01857a033
Walsh, R.; Wells, J. M. J. Chem. Soc., Perkin Trans. 2 1976, 52-55. DOI: https://doi.org/10.1039/P29760000052. DOI: https://doi.org/10.1039/P29760000052
Rowley, D.; Steiner, H. Discuss. Faraday Soc. 1951, 10, 198-213. DOI: https://doi.org/10.1039/DF9511000198. DOI: https://doi.org/10.1039/df9511000198
Smith, S. R.; Gordon, A. S. J. Phys. Chem. 1961, 65, 1124-1128. DOI: https://doi.org/10.1021/j100825a008. DOI: https://doi.org/10.1021/j100825a008
Skinner, J. L.; Sliepcevich, C. M. Ind. Eng. Chem. Fundam. 1963, 2, 168-172. DOI: https://doi.org/10.1021/i160007a002. DOI: https://doi.org/10.1021/i160007a002
Uchiyama, M.; Tomioka, T.; Amano, A. J. Phys. Chem. 1964, 68, 1878-1881. DOI: https://doi.org/10.1021/j100789a036. DOI: https://doi.org/10.1021/j100789a036
Van Sickle, D. E.; Rodin, J. O. J. Am. Chem. Soc. 1964, 86, 3091-3094. DOI: https://doi.org/10.1021/ja01069a024. DOI: https://doi.org/10.1021/ja01069a024
Tardy, D. C.; Ireton, R.; Gordon, A. S. J. Am. Chem. Soc. 1979, 101, 1508-1514. DOI: https://doi.org/10.1021/ja00500a024. DOI: https://doi.org/10.1021/ja00500a024
Houk, K. N.; Lin, Y. T.; Brown, F. K. J. Am. Chem. Soc. 1986, 108, 554-556. DOI: https://doi.org/10.1021/ja00263a059. DOI: https://doi.org/10.1021/ja00263a059
Burke, L. A.; Leroy, G.; Sana, M. Theor. Chem. Acc. 1975, 40, 313-321. DOI: https://doi.org/10.1007/bf00668337. DOI: https://doi.org/10.1007/BF00668337
Townshend, R. E.; Ramunni, G.; Segal, G.; Hehre, W. J.; Salem, L. J. Am. Chem. Soc. 1976, 98, 2190-2198. DOI: https://doi.org/10.1021/ja00424a031. DOI: https://doi.org/10.1021/ja00424a031
Burke, L. A.; Leroy, G. Theor. Chem. Acc. 1977, 44, 219-221. DOI: https://doi.org/10.1007/bf00549104. DOI: https://doi.org/10.1007/BF00549104
Jug, K.; Krüger, H. W. Theor. Chem. Acc. 1979, 52, 19-26. DOI: https://doi.org/10.1007/bf00581697. DOI: https://doi.org/10.1007/BF00581697
Bernardi, F.; Bottoni, A.; Robb, M. A.; Field, M. J.; Hillier, I. H.; Guest, M. F. J. Chem. Soc., Chem. Commun. 1985, 1051-1052. DOI: https://doi.org/10.1039/C39850001051. DOI: https://doi.org/10.1039/C39850001051
Dewar, M. J. S.; Olivella, S.; Stewart, J. J. P. J. Am. Chem. Soc. 1986, 108, 5771-5779. DOI: https://doi.org/10.1021/ja00279a018. DOI: https://doi.org/10.1021/ja00279a018
Bernardi, F.; Bottoni, A.; Field, M. J.; Guest, M. F.; Hillier, I. H.; Robb, M. A.; Venturini, A. J. Am. Chem. Soc. 1988, 110, 3050-3055. DOI: https://doi.org/10.1021/ja00218a009. DOI: https://doi.org/10.1021/ja00218a009
Bach, R. D.; McDouall, J. J. W.; Schlegel, H. B.; Wolber, G. J. J. Org. Chem. 1989, 54, 2931-2935. DOI: https://doi.org/10.1021/jo00273a029. DOI: https://doi.org/10.1021/jo00273a029
Houk, K. N.; Loncharich, R. J.; Blake, J. F.; Jorgensen, W. L. J. Am. Chem. Soc. 1989, 111, 9172-9176. DOI: https://doi.org/10.1021/ja00208a006. DOI: https://doi.org/10.1021/ja00208a006
Jorgensen, W. L.; Lim, D.; Blake, J. F. J. Am. Chem. Soc. 1993, 115, 2936-2942. DOI: https://doi.org/10.1021/ja00060a048. DOI: https://doi.org/10.1021/ja00060a048
Li, Y.; Houk, K. N. J. Am. Chem. Soc. 1993, 115, 7478-7485. DOI: https://doi.org/10.1021/ja00069a055. DOI: https://doi.org/10.1021/ja00069a055
Herges, R.; Jiao, H.; Schleyer, P. v. R. Angew. Chem., Int. Ed. 1994, 33, 1376-1378. DOI: https://doi.org/10.1002/anie.199413761. DOI: https://doi.org/10.1002/anie.199413761
Houk, K. N.; Li, Y.; Storer, J.; Raimondi, L.; Beno, B. J. Chem. Soc., Faraday Trans. 1994, 90, 1599-1604. DOI: https://doi.org/10.1039/FT9949001599. DOI: https://doi.org/10.1039/ft9949001599
Bernardi, F.; Celani, P.; Olivucci, M.; Robb, M. A.; Suzzi-Valli, G. J. Am. Chem. Soc. 1995, 117, 10531-10536. DOI: https://doi.org/10.1021/ja00147a014. DOI: https://doi.org/10.1021/ja00147a014
Jursic, B.; Zdravkovski, Z. J. Chem. Soc., Perkin Trans. 2 1995, 1223-1226. DOI: https://doi.org/10.1039/P29950001223. DOI: https://doi.org/10.1039/P29950001223
Goldstein, E.; Beno, B.; Houk, K. N. J. Am. Chem. Soc. 1996, 118, 6036-6043. DOI: https://doi.org/10.1021/ja9601494. DOI: https://doi.org/10.1021/ja9601494
Torrent, M.; Durán, M.; Solà, M. SCIENTIA gerundensis. 1996, 22, 123-131.
Branchadell, V. Int. J. Quantum Chem. 1997, 61, 381-388. DOI: https://doi.org/10.1002/(sici)1097-461x(1997)61:3<381::aid-qua3>3.0.co;2-s. DOI: https://doi.org/10.1002/(SICI)1097-461X(1997)61:3<381::AID-QUA3>3.3.CO;2-8
Jiao, H.; Schleyer, P. v. R. J. Phys. Org. Chem. 1998, 11, 655-662. DOI: https://doi.org/10.1002/(SICI)1099-1395(199808/09)11:8/9<655::AID-POC66>3.0.CO;2-U. DOI: https://doi.org/10.1002/(SICI)1099-1395(199808/09)11:8/9<655::AID-POC66>3.3.CO;2-L
Spino, C.; Pesant, M.; Dory, Y. Angew. Chem., Int. Ed. 1998, 37, 3262-3265. DOI: https://doi.org/10.1002/(sici)1521-3773(19981217)37:23<3262::aid-anie3262>3.0.co;2-t. DOI: https://doi.org/10.1002/(SICI)1521-3773(19981217)37:23<3262::AID-ANIE3262>3.0.CO;2-T
Bradley, A. Z.; Kociolek, M. G.; Johnson, R. P. J. Org. Chem. 2000, 65, 7134-7138. DOI: https://doi.org/10.1021/jo000916o. DOI: https://doi.org/10.1021/jo000916o
Sakai, S. J. Phys. Chem. A. 2000, 104, 922-927. DOI: https://doi.org/10.1021/jp9926894. DOI: https://doi.org/10.1021/jp9926894
Huang, C.-H.; Tsai, L.-C.; Hu, W.-P. J. Phys. Chem. A. 2001, 105, 9945-9953. DOI: https://doi.org/10.1021/jp012740f. DOI: https://doi.org/10.1021/jp012740f
Dinadayalane, T. C.; Vijaya, R.; Smitha, A.; Sastry, G. N. J. Phys. Chem. A. 2002, 106, 1627-1633. DOI: https://doi.org/10.1021/jp013910r. DOI: https://doi.org/10.1021/jp013910r
Guner, V.; Khuong, K. S.; Leach, A. G.; Lee, P. S.; Bartberger, M. D.; Houk, K. N. J. Phys. Chem. A. 2003, 107, 11445-11459. DOI: https://doi.org/10.1021/jp035501w. DOI: https://doi.org/10.1021/jp035501w
Lischka, H.; Ventura, E.; Dallos, M. ChemPhysChem 2004, 5, 1365-1371. DOI: https://doi.org/10.1002/cphc.200400104. DOI: https://doi.org/10.1002/cphc.200400104
Sakai, S. J. Phys. Chem. A. 2006, 110, 6339-6344. DOI: https://doi.org/10.1021/jp0560011. DOI: https://doi.org/10.1021/jp0560011
Hirao, H. J. Comput. Chem. 2008, 29, 1399-1407. DOI: https://doi.org/10.1002/jcc.20899. DOI: https://doi.org/10.1002/jcc.20899
Murray, J. S.; Yepes, D.; Jaque, P.; Politzer, P. Comput. Theor. Chem. 2015, 1053, 270-280. DOI: https://doi.org/10.1016/j.comptc.2014.08.010. DOI: https://doi.org/10.1016/j.comptc.2014.08.010
Scarborough, D. L. A.; Kobayashi, R.; Thompson, C. D.; Izgorodina, E. I. Int. J. Quantum Chem. 2015, 115, 989-1001. DOI: https://doi.org/10.1002/qua.24933. DOI: https://doi.org/10.1002/qua.24933
Sexton, T.; Kraka, E.; Cremer, D. J. Phys. Chem. A 2016, 120, 1097-1111. DOI: https://doi.org/10.1021/acs.jpca.5b11493. DOI: https://doi.org/10.1021/acs.jpca.5b11493
Domingo, L. R.; Ríos-Gutiérrez, M.; Pérez, P. Tetrahedron. 2017, 73, 1718-1724. DOI: https://doi.org/10.1016/j.tet.2017.02.012. DOI: https://doi.org/10.1016/j.tet.2017.02.012
Chakraborty, D.; Das, R.; Chattaraj, P. K. ChemPhysChem. 2017, 18, 2162-2170. DOI: https://doi.org/10.1002/cphc.201700308. DOI: https://doi.org/10.1002/cphc.201700308
Chen, B.; Hoffmann, R.; Cammi, R. Angew. Chem., Int. Ed. 2017, 56, 11126-11142. DOI: https://doi.org/10.1002/anie.201705427. DOI: https://doi.org/10.1002/anie.201705427
Casals-Sainz, J. L.; Francisco, E.; Martín Pendás, Á. Z. Anorg. Allg. Chem. 2020, 646, 1062-1072. DOI: https://doi.org/10.1002/zaac.202000038. DOI: https://doi.org/10.1002/zaac.202000038
Jara-Cortés, J.; Leal-Sánchez, E.; Hernández-Trujillo, J. J. Phys. Chem. A. 2020, 124, 6370-6379. DOI: https://doi.org/10.1021/acs.jpca.0c04171. DOI: https://doi.org/10.1021/acs.jpca.0c04171
Ayarde-Henríquez, L.; Guerra, C.; Duque-Noreña, M.; Rincón, E.; Pérez, P.; Chamorro, E. J. Phys. Chem. A. 2021, 125, 5152-5165. DOI: https://doi.org/10.1021/acs.jpca.1c01448. DOI: https://doi.org/10.1021/acs.jpca.1c01448
McLachlan, A. D.; Ball, M. A. Rev. Mod. Phys. 1964, 36, 844-855. DOI: https://doi.org/10.1103/RevModPhys.36.844. DOI: https://doi.org/10.1103/RevModPhys.36.844
Jurečka, P.; Šponer, J.; Černý, J.; Hobza, P. Phys. Chem. Chem. Phys. 2006, 8, 1985-1993. DOI: https://doi.org/10.1039/b600027d. DOI: https://doi.org/10.1039/B600027D
Grimme, S. J. Chem. Phys. 2003, 118, 9095-9102. DOI: https://doi.org/10.1063/1.1569242. DOI: https://doi.org/10.1063/1.1569242
Gerenkamp, M.; Grimme, S. Chem. Phys. Lett. 2004, 392, 229-235. DOI: https://doi.org/10.1016/j.cplett.2004.05.063. DOI: https://doi.org/10.1016/j.cplett.2004.05.063
Goumans, T. P. M.; Ehlers, A. W.; Lammertsma, K.; Würthwein, E.-U.; Grimme, S. Chem. - Eur. J. 2004, 10, 6468-6475. DOI: https://doi.org/10.1002/chem.200400250. DOI: https://doi.org/10.1002/chem.200400250
Piacenza, M.; Grimme, S. J. Comput. Chem. 2004, 25, 83-99. DOI: https://doi.org/10.1002/jcc.10365. DOI: https://doi.org/10.1002/jcc.10365
Grimme, S.; Mück-Lichtenfeld, C.; Würthwein, E.-U.; Ehlers, A. W.; Goumans, T. P. M.; Lammertsma, K. J. Phys. Chem. A 2006, 110, 2583-2586. DOI: https://doi.org/10.1021/jp057329x. DOI: https://doi.org/10.1021/jp057329x
Hill, J. G.; Platts, J. A.; Werner, H.-J. Phys. Chem. Chem. Phys. 2006, 8, 4072-4078. DOI: https://doi.org/10.1039/B608623C. DOI: https://doi.org/10.1039/b608623c
Antony, J.; Grimme, S. J. Phys. Chem. A. 2007, 111, 4862-4868. DOI: https://doi.org/10.1021/jp070589p. DOI: https://doi.org/10.1021/jp070589p
Takatani, T.; David Sherrill, C. Phys. Chem. Chem. Phys. 2007, 9, 6106-6114. DOI: https://doi.org/10.1039/B709669K. DOI: https://doi.org/10.1039/b709669k
Bates, D. M.; Anderson, J. A.; Oloyede, P.; Tschumper, G. S. Phys. Chem. Chem. Phys. 2008, 10, 2775-2779 DOI: https://doi.org/10.1039/B718720C. DOI: https://doi.org/10.1039/b718720c
Takatani, T.; Hohenstein, E. G.; Sherrill, C. D. J. Chem. Phys. 2008, 128, 124111-124117. DOI: https://doi.org/10.1063/1.2883974. DOI: https://doi.org/10.1063/1.2883974
King, R. A. Mol. Phys. 2009, 107, 789-795. DOI: https://doi.org/10.1080/00268970802641242. DOI: https://doi.org/10.1080/00268970802641242
Riley, K. E.; Platts, J. A.; Řezáč, J.; Hobza, P.; Hill, J. G. J. Phys. Chem. A. 2012, 116, 4159-4169. DOI: https://doi.org/10.1021/jp211997b. DOI: https://doi.org/10.1021/jp211997b
Frisch, M. J.; Trucks, G. W.; Schlegel, H. B.; Scuseria, G. E.; Robb, M. A.; Cheeseman, J. R.; Scalmani, G.; Barone, V.; Mennucci, B.; Petersson, G. A.; Nakatsuji, H.; Caricato, M.; Li, X.; Hratchian, H. P.; Izmaylov, A. F.; Bloino, J.; Zheng, G.; Sonnenberg, J. L.; Hada, M.; Ehara, M.; Toyota, K.; Fukuda, R.; Hasegawa, J.; Ishida, M.; Nakajima, T.; Honda, Y.; Kitao, O.; Nakai, H.; Vreven, T.; Montgomery, J., J. A.; Peralta, J. E.; Ogliaro, F.; Bearpark, M.; Heyd, J. J.; Brothers, E.; Kudin, K. N.; Staroverov, V. N.; Keith, T.; Kobayashi, R.; Normand, J.; Raghavachari, K.; Rendell, A.; Burant, J. C.; Iyengar, S. S.; Tomasi, J.; Cossi, M.; Rega, N.; Millam, J. M.; Klene, M.; Knox, J. E.; Cross, J. B.; Bakken, V.; Adamo, C.; Jaramillo, J.; Gomperts, R.; Stratmann, R. E.; Yazyev, O.; Austin, A. J.; Cammi, R.; Pomelli, C.; Ochterski, J. W.; Martin, R. L.; Morokuma, K.; Zakrzewski, V. G.; Voth, G. A.; Salvador, P.; Dannenberg, J. J.; Dapprich, S.; Daniels, A. D.; Farkas, O.; Foresman, J. B.; Ortiz, J. V.; Cioslowski, J.; Fox, D. J. Gaussian 09, Revision D.01, Gaussian, Inc.: Wallingford, CT, 2013.
Kesharwani, M. K.; Brauer, B.; Martin, J. M. L. J. Phys. Chem. A. 2015, 119, 1701-1714. DOI: https://doi.org/10.1021/jp508422u. DOI: https://doi.org/10.1021/jp508422u
Boys, S. F.; Bernardi, F. Mol. Phys. 1970, 19, 553-566. DOI: https://doi.org/10.1080/00268977000101561. DOI: https://doi.org/10.1080/00268977000101561
NIST Chemistry Webbook. http://webbook.nist.gov, accessed in June 2022.
Alvarez-Idaboy, J. R.; Galano, A. Theor. Chem. Acc. 2010, 126, 75-85. DOI: https://doi.org/10.1007/s00214-009-0676-z. DOI: https://doi.org/10.1007/s00214-009-0676-z
Xidos, J. D.; Poirier, R. A.; Pye, C. C.; Burnell, D. J. J. Org. Chem. 1998, 63, 105-112. DOI: https://doi.org/10.1021/jo9712815. DOI: https://doi.org/10.1021/jo9712815
Houk, K. N.; Gandour, R. W.; Strozier, R. W.; Rondan, N. G.; Paquette, L. A. J. Am. Chem. Soc. 1979, 101, 6797-6802. DOI: https://doi.org/10.1021/ja00517a001. DOI: https://doi.org/10.1021/ja00517a001
Coxon, J. M.; Grice, S. T.; Maclagan, R. G. A. R.; McDonald, D. Q. J. Org. Chem. 1990, 55, 3804-3807. DOI: https://doi.org/10.1021/jo00299a021. DOI: https://doi.org/10.1021/jo00299a021
Ess, D. H.; Houk, K. N. J. Am. Chem. Soc. 2007, 129, 10646-10647. DOI: https://doi.org/10.1021/ja0734086. DOI: https://doi.org/10.1021/ja0734086
Ess, D. H.; Houk, K. N. J. Am. Chem. Soc. 2008, 130, 10187-10198. DOI: https://doi.org/10.1021/ja800009z. DOI: https://doi.org/10.1021/ja800009z
Jones, G. O.; Houk, K. N. J. Org. Chem. 2008, 73, 1333-1342. DOI: https://doi.org/10.1021/jo702295d. DOI: https://doi.org/10.1021/jo702295d
Hayden, A. E.; Houk, K. N. J. Am. Chem. Soc. 2009, 131, 4084-4089. DOI: https://doi.org/10.1021/ja809142x. DOI: https://doi.org/10.1021/ja809142x
Bickelhaupt, F. M.; Houk, K. N. Angew. Chem., Int. Ed. 2017, 56, 10070-10086. DOI: https://doi.org/10.1002/anie.201701486. DOI: https://doi.org/10.1002/anie.201701486
Bickelhaupt, F. M. J. Comput. Chem. 1999, 20, 114-128. DOI: https://doi.org/10.1002/(SICI)1096-987X(19990115)20:1<114::AID-JCC12>3.0.CO;2-L. DOI: https://doi.org/10.1002/(SICI)1096-987X(19990115)20:1<114::AID-JCC12>3.0.CO;2-L
Diefenbach, A.; Bickelhaupt, F. M. J. Phys. Chem. A 2004, 108, 8460-8466. DOI: 10.1021/jp047986+. DOI: https://doi.org/10.1021/jp047986+
De Jong, G. T.; Bickelhaupt, F. M. ChemPhysChem 2007, 8, 1170-1181. DOI: https://doi.org/10.1002/cphc.200700092. DOI: https://doi.org/10.1002/cphc.200700092
Van Zeist, W.-J.; Bickelhaupt, F. M. Org. Biomol. Chem. 2010, 8, 3118-3127. DOI: https://doi.org/10.1039/B926828F. DOI: https://doi.org/10.1039/b926828f
Fernández, I.; Bickelhaupt, F. M. Chem. Soc. Rev. 2014, 43, 4953-4967. DOI: https://doi.org/10.1039/C4CS00055B. DOI: https://doi.org/10.1039/C4CS00055B
Wolters, L. P.; Bickelhaupt, F. M. Wiley Interdiscip. Rev.: Comput. Mol. Sci. 2015, 5, 324-343. DOI: https://doi.org/10.1002/wcms.1221. DOI: https://doi.org/10.1002/wcms.1221
Eisler, B.; Wassermann, A. Discuss. Faraday Soc. 1951, 235. DOI: https://doi.org/10.1039/DF9511000213. DOI: https://doi.org/10.1039/df9511000213
Eisler, B.; Wassermann, A. J. Chem. Soc. 1953, 979-982. DOI: https://doi.org/10.1039/JR9530000979. DOI: https://doi.org/10.1039/JR9530000979
Craig, D.; Shipman, J. J.; Fowler, R. B. J. Am. Chem. Soc. 1961, 83, 2885-2891. DOI: https://doi.org/10.1021/ja01474a023. DOI: https://doi.org/10.1021/ja01474a023
Morokuma, K. J. Chem. Phys. 1971, 55, 1236-1244. DOI: https://doi.org/10.1063/1.1676210. DOI: https://doi.org/10.1063/1.1676210
Kitaura, K.; Morokuma, K. Int. J. Quantum Chem. 1976, 10, 325-340. DOI: https://doi.org/10.1002/qua.560100211. DOI: https://doi.org/10.1002/qua.560100211
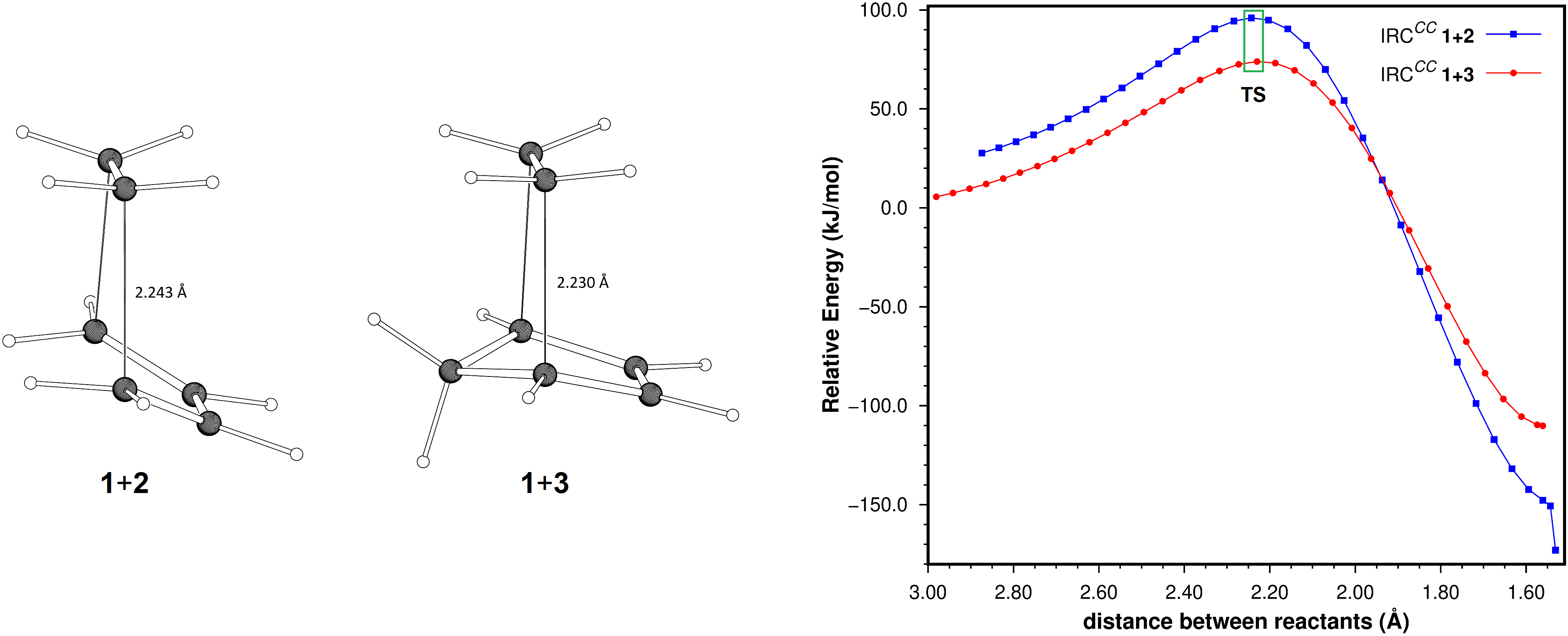
Downloads
Additional Files
Published
Issue
Section
License
Copyright (c) 2023 Dr. Hugo Alejandro Jiménez Vázquez, M. en C. Luis Almazán Sánchez, Dra. Adriana Benavides Macias

This work is licensed under a Creative Commons Attribution-NonCommercial 4.0 International License.
Authors who publish with this journal agree to the following terms:
- Authors retain copyright and grant the journal right of first publication with the work simultaneously licensed under a Creative Commons Attribution License that allows others to share the work with an acknowledgement of the work's authorship and initial publication in this journal.
- Authors are able to enter into separate, additional contractual arrangements for the non-exclusive distribution of the journal's published version of the work (e.g., post it to an institutional repository or publish it in a book), with an acknowledgement of its initial publication in this journal.
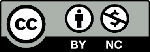