Surface Characteristics of Different Wood and Coal-Based Activated Carbons for Preparation of Carbon Molecular Sieve
DOI:
https://doi.org/10.29356/jmcs.v63i2.666Keywords:
Activated Carbon, Surface Areas, Pore Volume, Size Reduction, Carbon Molecular SieveAbstract
Abstract. In this study, four different nanostructures precursors, pistachio wood, walnuts wood, forest wood and coal (Anthracite) were selected to produce activated carbon. The experiments were done in industrial scale using a rotary furnace with temperature between 850 and 920 ºC for 45 minutes followed by steam. The product was grounded and divided in three particle sizes, 8x30, 30x50, and 60x80 meshes. The physical properties and surface chemistry of the activated carbon samples were determined by imaging of BET-N2 adsorption. The results obtained from measurements of iodine and methylene blue numbers, surface area, pore volume and comparison of surface area and pore volume, show that by decreasing of particle sizes of each sample, increase the surface area and micro pore volumes significantly. The magnitude of changes in surface area with particle size is a significant factor in defining the effectiveness and suitability of an activated carbon for the production of carbon molecular sieve. Our results indicate that the highest pore volume belongs to pistachio wood, which was increased from 0.168 to 0.271 cm3/g from 8x30 to 60x80 mesh.
Resumen. En este estudio, se seleccionaron cuatro precursores de nanoestructuras diferentes, madera de pistache, madera de nueces, madera de bosque y carbón (antracita) para producir carbón activado. Los experimentos se realizaron a escala industrial utilizando un horno rotatorio con una temperatura entre 850 y 920 ºC durante 45 minutos, seguido de vapor. El producto se molió y se dividió en tres tamaños de partículas, en mallas de 8x30, 30x50 y 60x80. Las propiedades físicas y la química de la superficie de las muestras de carbón activado se determinaron mediante imágenes de la adsorción de BET-N2. Los resultados obtenidos de las mediciones de los números de yodo y azul de metileno, área de superficie, volumen de poros y comparación de área de superficie y volumen de poros muestran que, al disminuir los tamaños de partícula de cada muestra, aumenta significativamente el área de superficie y los volúmenes de microporos. La magnitud de los cambios en el área de la superficie con el tamaño de partícula es un factor significativo en la definición de la efectividad y la idoneidad de un carbón activado para la producción de tamiz molecular de carbono. Nuestros resultados indican que el mayor volumen de poros pertenece a la madera de pistache, que aumentó de la malla de 0.168 a 0.271 cm3/g de 8x30 a 60x80.
Downloads
References
C. Stiehler; F. Calaza; W.-D. Schneider; N. Nilius; H.-J. Freund. Phys. Rev. Lett., 115, 2015, 036804.
M. Thommes; K. Kaneko; A.V. Neimark; J.P. Olivier; F. Rodriguez-Reinoso; J. Rouquerol; K.S. Sing. Pure Appl. Chem., 2015, 87, 1051-1069.
S. Fatemi; M. Foroutan. Int. J. Environ. Sci. Technol., 2016, 13, 457-470.
Z.-Y. Li; M.S. Akhtar; D.-H. Kwak; O.-B. Yang. Appl. Surf. Sci., 2017, 404, 88-93.
J. Rouquerol; F. Rouquerol; P. Llewellyn; G. Maurin; K.S. Sing. Adsorption by powders and porous solids: principles, methodology and applications, Academic press (2013).
S. Li; K. Han; J. Li; M. Li; C. Lu. Microporous Mesoporous Mater, 2017, 243, 291-300.
Q. Wang; J. Luo; Z. Zhong; A. Borgna. Energy Environ. Sci., 2011, 4, 42-55.
A. Samanta; A. Zhao; G.K. Shimizu; P. Sarkar; R. Gupta. Ind. Eng. Chem. Res., 2011, 51, 1438-1463.
E. Alt?nt?g; H. Altundag; M. Tuzen; A. Sar?. Chem. Eng. Res. Des., 2017, 151-163.
S.M. Fatemi; Z. Abbasi; H. Rajabzadeh; S.A. Hashemizadeh; A.N. Deldar. Eur. Phys. J. D, 2017, 71, 194.
Z. Chen; S. Deng; H. Wei; B. Wang; J. Huang; G. Yu. Environ. Sci. Eng., 2013, 7, 326-340.
F. Mohammadnezhad; M. Feyzi; S. Zinadini. J. Ind. Eng. Chem., 2019, 71, 99-111.
M.H. El-Naas; M.A. Alhaija; S. Al-Zuhair. Environ. Sci. Pollut. Res., 2017, 24, 7511-7520.
R.V. Siriwardane; M.-S. Shen; E.P. Fisher; J.A. Poston. Energy & Fuels, 2001, 15, 279-284.
S. Brunauer; P.H. Emmett; E. Teller. J. Am. Chem. Soc., 1938, 60, 309-319.
B. Panella; M. Hirscher; S. Roth. Carbon, 2005, 43, 2209-2214.
P. Nugent; Y. Belmabkhout; S.D. Burd; A.J. Cairns; R. Luebke; K. Forrest; T. Pham; S. Ma; B. Space; L. Wojtas. Nature, 2013, 495, 80.
H. Kim; Y. Kim; M. Yoon; S. Lim; S.M. Park; G. Seo; K. Kim. J. Am. Chem. Soc., 2010, 132, 12200-12202.
K.M. Steel; W.J. Koros. Carbon, 2003, 41, 253-266.
M. Thommes; K. Kaneko; A.V. Neimark; J.P. Olivier; F. Rodriguez-Reinoso; J. Rouquerol; K.S. Sing. Pure Appl. Chem., 2015, 87, 1051-1069.
J. Kipling; R. Wilson. J. Applied Chem., 1960, 10, 109-113.
B.R. Puri; R. Bansal. Carbon, 1965, 3, 227-230.
S. Ummartyotin; H. Manuspiya. Renewable Sustainable Energy Rev., 2015, 41, 402-412.
E. Köseo?lu; C. Akmil-Ba?ar. Adv. Powder Technol., 2015, 26, 811-818.
M.A. Yahya; Z. Al-Qodah; C.Z. Ngah. Renewable Sustainable Energy Rev., 2015, 46, 218-235.
Á. Simay; L.G. Nagy; A. Bóta. Chem. Eng., 1984, 28, 293-297.
N. Letaief; A. Lucas-Girot; H. Oudadesse; R. Dorbez-Sridi. J. Biosci. Med., 2014, 2, 57.
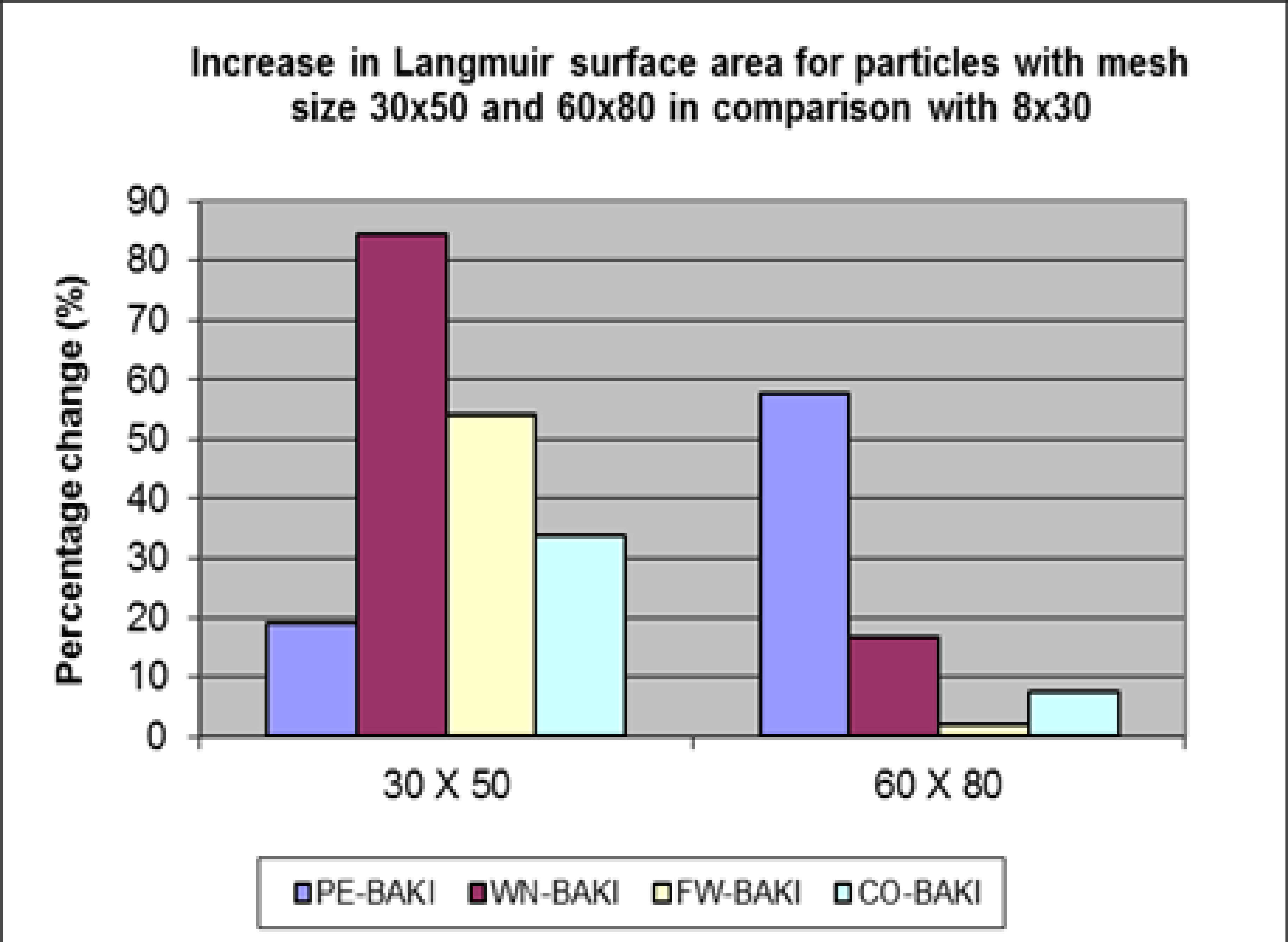
Downloads
Published
Issue
Section
License
Authors who publish with this journal agree to the following terms:
- Authors retain copyright and grant the journal right of first publication with the work simultaneously licensed under a Creative Commons Attribution License that allows others to share the work with an acknowledgement of the work's authorship and initial publication in this journal.
- Authors are able to enter into separate, additional contractual arrangements for the non-exclusive distribution of the journal's published version of the work (e.g., post it to an institutional repository or publish it in a book), with an acknowledgement of its initial publication in this journal.
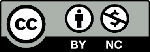